What are Ethers?
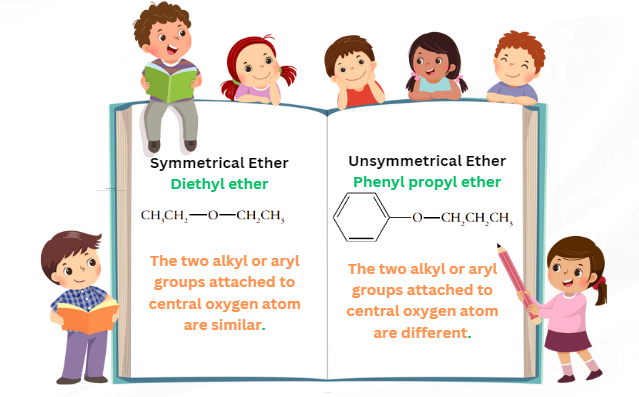
Before coming to williamson ether synthesis look at ethers first. Similar to ketones, ethers belong to a class of organic compounds, having a central oxygen atom bonds to two alkyl or aryl groups. Based on these two groups (alkyl/aryl), ethers can be classified as symmetrical or unsymmetrical ethers.
Symmetrical ethers: if the two alkyl or aryl groups attached to the central oxygen atom are similar, the ether is symmetrical. The example includes diethyl ether.
Unsymmetrical ethers: if the two alkyl or aryl groups attached to the central oxygen atom are different, the ether is unsymmetrical. Examples include phenyl propyl ether.
Naturally occurring Ethers.
We know that every organic compound is organic because it exists in nature. Similarly, ethers are naturally occuring compounds. A key active component of marijuana is tetrahydrocannabinol (THC), which is a six-membered cyclic ether. Similarly, morphine contains five-membered ether rings, while three-membered (epoxide) cyclic ethers are rare in nature.
Interestingly, the juvenile hormone of some insects have cis-substituted epoxide. This compound primarily participates in the maturation of insects.
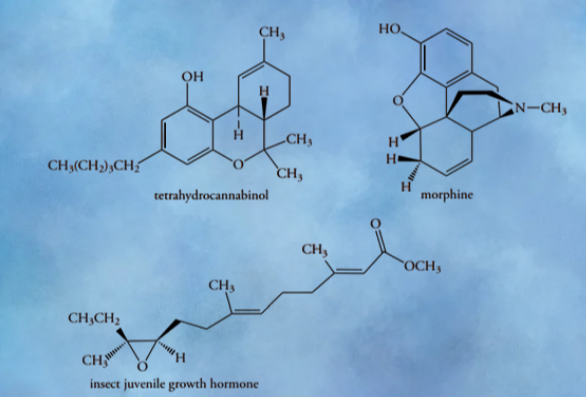
The commercial demand for naturally occurring compounds is very high to form tons of products for human benefit in health, economic, industrial, and educational sectors, etc. Therefore, due to the high demand for organic compounds, these compounds and derivatives can be prepared in laboratories and industries on a large scale, and a huge variety of reaction mechanisms are involved in the synthesis of various class of organic compounds and their derivatives. The synthetic method particulary use for ethers is Williamson Ether synthesis.
What is Williamson Ether Synthesis?
Whenever you think of how to make ether, remember the most convenient way of ether formation is the Williamson synthesis of ether, also known as Williamson etherification.
Alexander Williamson, an English scientist, developed a protocol to synthesize ether in 1850, and the reaction was named after him as Williamson ether synthesis.
It involves the reaction between a deprotonated alcohol (an alkoxide) and an alkyl halide to form an ether.
The general reaction equation for a Williamson ether synthesis is:

In a specific Williamson synthesis, ethers can be form by the reaction of metal alkoxide, which displaces a halide ion from alkyl halide. In the initial step, the alkoxide ion is usually prepare by reacting an alcohol with a strong base like NaH or NaOH. One example of Williamson ether synthesis is the reaction between chloroethane and sodium ethoxide to form diethyl ether.
C2H5Cl + C2H5ONa → C2H5O C2H5 + NaCl
Another Williamson synthesis of ether involves the reaction between cyclopentanol and methyl bromide.
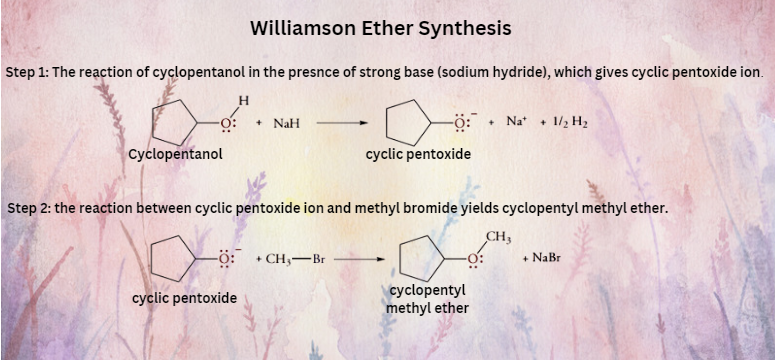
So, the Williamson ether synthesis involves the treatment of a haloalkane with a metal alkoxide.
Conditions For Williamson’s Ether Synthesis.
The Williamson synthesis of ethers involves a bimolecular nucleophilic substitution (SN2) mechanism between a primary alkyl halide and an alkoxide (i.e. the conjugate base of alcohol, RO)–.
In an SN2 reaction, the Williamson synthesis gives the best yield with primary alkyl halide as the reaction proceeds with the displacement of halide ions as a leaving group.
The yield is low for the secondary alkyl halide because they proceed with an elimination reaction alongside SN2 when reacting with an alkoxide ion. Therefore, Williamson’s synthesis of ether from secondary alkyl halide gives a mixture of substitution and elimination products. The yield is not possible for tertiary alkyl halides as tertiary alkyls undergo elimination rather than substitution reaction.
The alkoxide or aryloxide may be primary, secondary, or tertiary depending on the alcohol undergoing deprotonation during a reaction with strong bases like sodium hydride (NaH) and potassium hydride (KH). The alcohol that provides the electron-rich alkoxide is often use as the solvent.
Now, the question may arise in your mind: why does alcohol not carry on the reaction? The answer is simple: the Sn2 reaction between alcohol and alky halides tends to be very slow because alcohols are comparatively poor nucleophiles. Therefore, alkoxide (the conjugate base of alcohol) is a better nucleophile to be use in the reaction. The alcohol that provides the electron-rich alkoxide is often utilize as a solvent (e.g. NaOEt in EtOH).
Thus, for synthesizing unsymmetrical ether with primary or tertiary alkyl groups, a primary alkyl halide and a tertiary alkoxide ion are the reagents of choice.
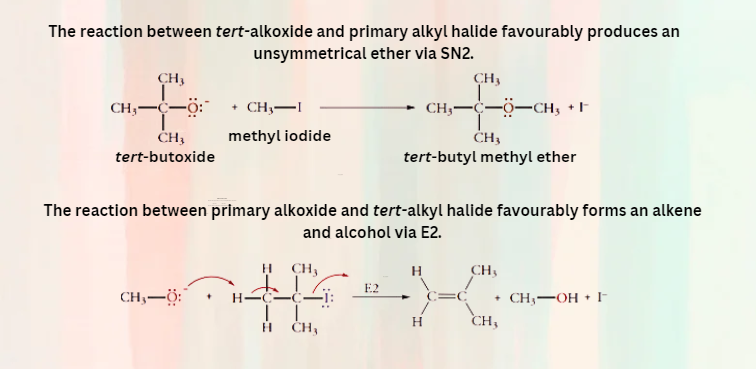
The above example clarifies which of the following reactions will provide the best yield of ether by Williamson ether synthesis. The tert-alkyl halide followed elimination to give alkene as a product.
Williamson Ether Synthesis Mechanism.
As discussed earlier, Williamson’s ether synthesis is a substitution reaction in which the bond formation and bond breaking occur simultaneously on the same carbon. During the Williamson substitution reaction, a bond breaks between carbon and the leaving group (typically a halide), and a new bond forms between carbon and oxygen.
First, the formation of alkoxide nucleophile, alcohol is treated with Sodium hydroxide (NaH) to form a conjugate base sodium alkoxide. The hydrogen releases as a by-product during the reaction.
Secondly, the nucleophile (alkoxide ion) is bond to a carbon atom as soon as the leaving group (halide group) leaves the carbon atom, making it a carbocation for a very short interval of time. Due to this, ether is form as a product.
Williamson Ether Synthesis: Stereochemistry:
We need to look at its stereochemistry deeply to identify the mechanism for the Williamson ether synthesis.
The SN2 (nucleophilic substitution bimolecular) reaction always proceeds in one step, i.e., the attack of the nucleophile and departure of the leaving group simultaneously occurs. When the carbon and the leaving group break, and a new bond forms between nucleophile and carbon, in the transition state.
The key step in the Williamson ether synthesis reaction involves the attack of nucleophiles from the backside on an alkyl halide. This is why primary alkyl halide gives better results: there is no steric hindrance on an alkyl group for the nucleophile to attack. Hence, the reaction is fastest with a primary alkyl halide. Secondary alkyl halide may give a better yield of ether. Still, they also prefer to undergo an elimination reaction, whereas Williamson ether synthesis is almost impossible with tert-alkyl halide due to high steric hindrance.
In the transition state of the reaction, carbon has penta co-ordinated geometry due to the partial bonding of carbon with both nucleophile and leaving group (i.e. where the bond between carbon and halide atom is breaking at the same time a bond between carbon and oxygen atom of alkoxide ion is building), which is shown by dotted lines.
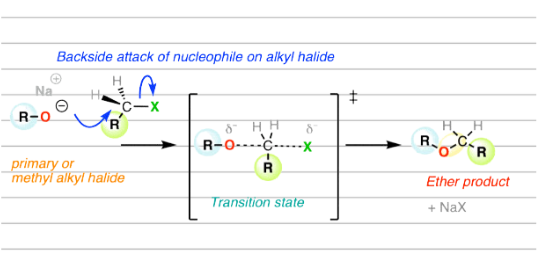
As the reaction proceeded, the bond between carbon and nucleophile strengthened. At the same time, the bond between carbon and the leaving group weakens, unless the bond breaks completely. Furthermore, due to the attack and bonding of nucleophiles from the backside, the product is formed. The geometry of product is inversion of configuration. This means the product’s geometry is inverted than the reactant’s.
Intramolecular Williamson Ether Synthesis.
We have now discussed aliphatic ethers. Actually the SN2 reaction of halogen-substituted alcohol can also form cyclic ethers, where halide and alcohol groups are present in the same compound.
Let’s take an example of bromo alcohol, more specifically, 5-bromo-penten-1-ol. A strong base like NaOH deprotonates the alcohol, forming bromo alkoxide. The intramolecular displacement of bromine, followed by the attack of alkoxide ion, acts as a nucleophile, resulting in a cyclic ether.
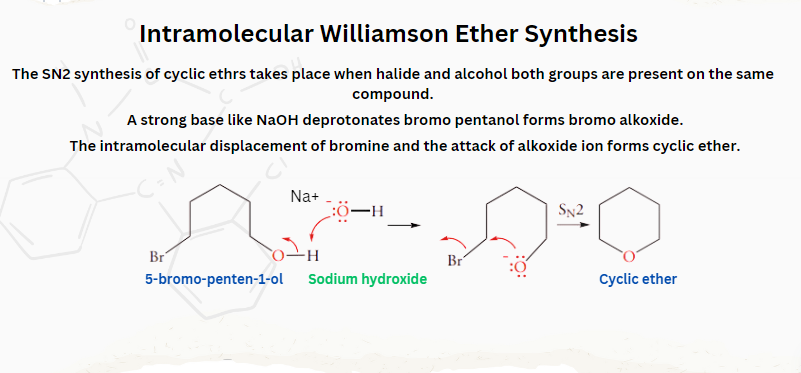
Now comes the question of whether chain size will affect ring formation. The answer is yes, considering the entropy factor (i.e. larger rings have more entropy, making them unfavourable due to larger ordering). With the increase in the number of atoms in the chain, the number of chain conformations and degree of freedom increases. Whereas, to form a suitable conformation for intramolecular reactions, the flexibility of a chain must be restricted. Therefore, the order of cyclization is:
3 > 4 > 5 > 6 > 7 > 8
With a small number of atoms, the two reacting centres lie nearby of each other, and the reaction is entropically favoured. As the number of atoms increases, the two reacting groups lie far away, making cyclization difficult.
Summarizing The Concept.
- For a Williamson synthesis of ether, the most favourable reagent is a primary alkyl halide, which sterically favours the attack of nucleophile (the alkoxide ion) from the backside. Sec- alkyl halide is a marginal starting reagent for Williamson ether synthesis. They equally tend to proceed with elimination to give alkenes.
- Don’t forget the feasibility of Williamson synthesis gained by using alkoxide nucleophile with its parent alcohol as a solvent. If you try to use alcohol as a nucleophile, the reaction would be very slow. Because alcohols are very poor nucleophiles.
- Read the above point again where I said “parent alcohol”. Because to get a desired product, for instance, diethyl ether, you should treat ethyl halide with ethoxide ion in ethanol. Instead, suppose you use ethoxide ion in a solvent propanol. In that case, the ethoxide ion undergoes an acid-base reaction with propanol to form ethanol and propoxide ion. This is then proceeds with ethyl halide and gives isopentyl propyl ether (an undesired product) instead of diethyl ether. See the image below.
- Suppose you are still wondering why the solvent in the Williamson ether synthesis normally conjugates acids of the alkoxide. It is because using alcohol as a solvent, which is not a conjugate acid of the alkoxide. It will lead to the undesired product yield. It is due to the excess amount of solvent in the reaction. Therefore, desired or parent alcohol as a solvent is recommended.
- Suppose you don’t want to use alcohol to generate alkoxide. In that case, you can use a hydride ion (H-). Moreover, by using sodium hydride (NaH) or potassium hydride (KH) added to the starting alcohol to get alkoxide. During this reaction, hydrogen gas formed as a by-product.
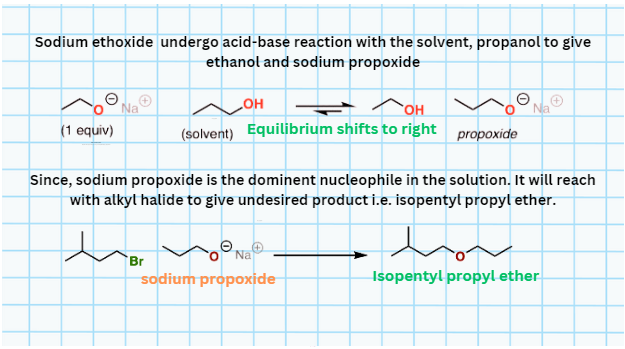
You May Want to Know:
Q.No.1 Which of the following ethers cannot be synthesized using Williamson ether synthesis?
The answer is di-tert– butyl ether, which can be formed due to the reaction between sodium tert-butoxide must be reacted with tert – butyl bromide. Since tert-alkyl halide undergoes an elimination reaction, the resultant product would be Isobutylene, not di-tert– butyl ether.
Q. No. 2 Provide the reagents necessary to prepare the following compound using a Williamson ether synthesis.
Methyl tert-butyl ether.
We discussed earlier that the best reactant combination for Williamson synthesis of ether is primary alkyl halide and tert– alkoxide ion. Accordingle, for the compound mentioned above, the best reagents are alkyl bromide and sodium tert– butoxide (NaOC(CH3)3).
Q. No. 3 Why do phenol groups make particularly good candidates for the Williamson ether synthesis?
The answer is simple. Usually, phenols undergo Williamson etherification similar to alcohols. Unlike the formation of alkoxide (the conjugate base of alcohol), it requires a strong base like sodium hydride (NaH). The conjugate base of phenol, is phenoxide. It can be prepared using simple hydroxide ion that acts as nucleophile in Williamson ether synthesis reaction.
Q. No. 4 Show the reaction of Williamson’s synthesis of cyclohexyl methyl ether.
The best Williamson synthesis of cyclohexyl methyl ether involves the following reaction:

References.
Ouellette, R. J., & Rawn, J. D. (2018). Ethers and Epoxides. In Organic Chemistry (pp. 507–536). Elsevier. https://doi.org/10.1016/b978-0-12-812838-1.50017-7
Ouellette, R. J., & Rawn, J. D. (2015). Aryl Halides, Phenols, and Anilines. In Organic Chemistry Study Guide (pp. 495–519). Elsevier. https://doi.org/10.1016/b978-0-12-801889-7.00024-8.
[…] shared pair completes the valance shell of each atom. More examples includes the type of bonding in ethers, ketone, phenols […]